Characterization of carbapenem resistant acinetobacter baumannii isolated from intensive care units in two teaching hospitals from Algeria and Tunisia
Sabrina Amiri, Samia Hammami, Kamel Amoura, Mazouz Dekhil, Ilhem Boutiba-Ben Boubaker
Corresponding author: Sabrina Amiri, Laboratory of Microbiology, University Hospital of Annaba, Algeria
Received: 25 Apr 2016 - Accepted: 14 Aug 2017 - Published: 12 Sep 2017
Domain: Other
Keywords: A. baumannii, Carbapenem resistance, OXA-23-like, Pulsed-field gel electrophoresis
©Sabrina Amiri et al. Pan African Medical Journal (ISSN: 1937-8688). This is an Open Access article distributed under the terms of the Creative Commons Attribution International 4.0 License (https://creativecommons.org/licenses/by/4.0/), which permits unrestricted use, distribution, and reproduction in any medium, provided the original work is properly cited.
Cite this article: Sabrina Amiri et al. Characterization of carbapenem resistant acinetobacter baumannii isolated from intensive care units in two teaching hospitals from Algeria and Tunisia. Pan African Medical Journal. 2017;28:19. [doi: 10.11604/pamj.2017.28.19.9713]
Available online at: https://www.panafrican-med-journal.com//content/article/28/19/full
Original article 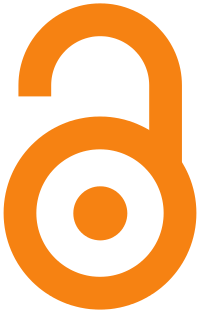
Characterization of carbapenem resistant acinetobacter baumannii isolated from intensive care units in two teaching hospitals from Algeria and Tunisia
Characterization of carbapenem resistant Acinetobacter baumannii isolated from intensive care units in two teaching hospitals from Algeria and Tunisia
Sabrina Amiri1,&, Samia Hammami2, Kamel Amoura1, Mazouz Dekhil1, Ilhem Boutiba-Ben Boubaker2,3
1Laboratory of Microbiology, University Hospital of Annaba, Algeria, 2Laboratory of Antimicrobial Resistance, Faculty of Medicine of Tunis-Tunisia University of Tunis El Manar, Tunisie, 3Laboratory of Microbiology of Charles Nicolle Hospital, Boulevard April 9, 1006 Tunis, Tunisia
&Corresponding author
Sabrina Amiri, Laboratory of Microbiology, University Hospital of Annaba, Algeria
Introduction: this study was conducted to identify the enzymatic mechanism of carbapenem resistance in A. baumannii isolated from intensive care units of 2 teaching hospitals (Charles Nicolle hospital of Tunis and University hospital of Annaba).
Methods: twenty seven non repetitive carbapenem-resistant A. baumannii were collected (7 strains in Algeria and 20 in Tunisia). Antibiotic susceptibility was performed by disk diffusion method. MICs were determined by agar dilution method. EDTA-disk synergy test was performed for metallo-β-lactamases (MBL) phenotypic detection. Detection of blaOXA-23-like, blaOXA-24-like, blaOXA-51-like and blaOXA-58-like families was performed by PCR followed by sequencing. Genetic relatedness between strains was investigated by pulsed-field gel electrophoresis (PFGE).
Results: strains were recovered especially from respiratory tract specimens (n=12) and blood (n=11). All strains were co-resistant to all β-lactams, gentamicin, amikacin and ciprofloxacin, but remainded susceptible to colistin. MBL production was negative for all isolates. blaOXA-51-like was detected in all strains and blaOXA-23-like in 23 strains. However, blaOXA-58-like and blaOXA-24-like were not found in any isolate. Six major PFGE patterns were found in the Tunisian isolates. However, the Algerian strains were clustered in one clone.
Conclusion: this study shows a high distribution of blaOXA-23 in imipenem-resistant A. baumannii isolated in Tunisia and Algeria. It demonstrated the epidemic diffusion of this multidrug resistant pathogen. Thus, strengthening of prevention measures are required to control further spread of carbapenemases in the two countries.
Acinetobacter baumannii has emerged as a leading cause of nosocomial infections, particularly among critically ill patients in intensive care units [1,2] A. baumannii clinical isolates are commonly resistant to multiple antimicrobial drug classes and have the ability to survive in the environment for prolonged periods of time, which facilitates their persistence in hospitals and make them a frequent cause of hospital outbreaks and an endemic healthcare associated pathogens [3]. Carbapenems have been widely used to treat these infections [4], but a trend of increasing resistance to this antibiotic class has been reported worldwide [5]; limiting drastically the range of therapeutic alternatives. Carbapenem resistance in A. baumannii results mainly from beta-lactamases production [6,7]. Metallo-beta-lactamases are prevalent in East Asia and Western Europe and confer resistance to all beta-lactams except aztreonam [1]. However, the OXA-type carbapenemases have emerged as the most widespread beta-lactamases with carbapenemase activity [8,9]. These enzymes can be sub-devised into 5 distinct groups: intrinsic OXA-51-like and acquired, OXA-23-like (OXA-23, OXA-27 and OXA-49), OXA-24-like (OXA-24, OXA-25, OXA-26, OXA-40 and OXA-72), OXA-58-like and OXA-143 [10-13]. In addition to beta-lactamases, carbapenem resistance in A. baumannii may also result from porin or penicillin-binding protein modifications [5]. The aim of this study was to determine the enzymatic mechanism of carbapenem resistance in A. baumannii isolates recovered from intensive care units of two teaching hospitals (Tunisia and Algeria) and to characterize nosocomial outbreaks by antibiotyping and pulsed-field gel electrophoresis (PFGE).
Bacterial isolates: this study analyzed 27 unduplicated carbapenem-resistant A. baumannii clinical isolates, collected from 2 intensive care units of 2 teaching hospitals [Charles Nicolle hospital of Tunis, (n= 20) and the university hospital of Annaba (n=7)], during 2009. Strains were isolated from respiratory tract specimens (n=12), blood (n=11), material (n=3) and urine (n=1) of 27 different patients aged from 17 to 78 years; 21 males and 6 females (sex ratio 3.3). Demographic and clinical characteristics of all patients were shown in Table 1. Strain identification was performed by conventional techniques and confirmed by PCR amplification of the endogen blaOXA-51-like gene [14].
Antimicrobial susceptibility testing: antibiotic susceptibility testing was performed using the disk diffusion method on Mueller Hinton agar. The minimal inhibitory concentrations (MICs) values of imipenem, meropenem, ticarcillin, ticarcillin /clavulanic acid; ceftazidime, cefepime and aztreonam were determined by the agar dilution technique. Current quality control testing was performed using the following organisms: E. coli ATCC 25922 and P. Aeruginosa ATCC 27853. The interpretation of the results was referred to the guidelines defined by the Clinical and Laboratory Standards Institute [3].
Screening for MBL-producing strains: detection of MBL was done by double disk synergy test using an imipenem disk placed 10 mm from a disk saturated with 5µl of EDTA (0.5 M pH 8). An enlargement of the inhibition zone of imipenem facing the disk of EDTA was considered as a positive test [15].
PCR amplification and sequencing: blaOXA-24-like, blaOXA-23-like and blaOXA-58-like genes were detected by PCR simplex assays as previously described [16]. DNA sequencing was performed by the dideoxy chain terminator method with Big Dye Terminator v.3.1 Cycle Sequencing Kit (Applied Biosystems, Foster City, CA) and analyzed using an ABI Prism 3100 genetic analyser (Applied Biosystems). Similarity searches and alignments of the nucleotide sequences were performed with the BLAST program (http://www.ncbi.nlm.nih.gov/BLAST).
Pulsed-field gel electrophoresis (PFGE): molecular typing of isolates was carried out, as described previously by Pulsed-Field Gel Electrophoresis (PFGE) using ApaI (Invitrogen) restriction endonuclease [14]. The ApaI restriction profiles were compared by visual inspection according to the criteria proposed by Tenover et al [17].
All strains were co-resistant to all β-lactams, gentamicin, amikacin, ciprofloxacin but remained susceptible to colistin. They showed high level of resistance to ticarcillin (MIC50>2048 μg/mL), ticarcillin-clavulanic acid (MIC50 >1024 μg/mL), aztreonam (MIC50 ≥256 μg/mL), ceftazidim (MIC50=2048 μg/mL), imipenem (MIC50= 256 μg/mL), meropenem (MIC50=128 μg/mL) and cefepime (MIC50=128 μg/mL) (Table 2). All strains showed a negative EDTA disk synergy test. PCR experiments for the naturally-occurring blaOXA-51-like gene gave positive results for all strains tested. The blaOXA-23-like was present in 23 strains (5 from Algeria and 18 from Tunisia) and the sequencing of the amplified fragments confirmed the presence of bla OXA-23 in all isolates, whereas no isolate harbored blaOXA-24-like or blaOXA-58-like genes. Six major PFGE patterns were found in the Tunisian isolates; named from A to F (Figure 1B). The genotype A was the most prevalent (12 strains) with 3 pulsotypes A1 (n=8), A2 (n=3) and A3 (n=1). However, the Algerian strains were clustered in one pattern G (Figure 1A).
A. baumannii has been stealthily gaining ground as an agent of serious nosocomial infections, including bacteremia, pneumonia, urinary tract and wound infections [18]. Historically, it has been associated with opportunistic infections; the last two decades have seen an increase in both the incidence and seriousness of A. baumannii infection, with the main targets being immunocompromized patients in intensive-care units [19]. The increase in A. baumannii infections has paralleled the alarming development of resistance [20]. Actually, multidrug-resistant A. baumannii is recognized to be among the most difficult antimicrobial-resistant Gram-negative bacilli to control and treat, especially if isolates are resistant to the carbapenem class of antimicrobial agents [21]. This study aimed to investigate the enzymatic mechanism of 27 carbapenem resistant A. baumannii clinical strains causing nosocomial infections in 27 debilitated patients hospitalized in two intensive care units of 2 different hospitals. As it was previously reported they mainly caused pneumonia and bacteremia [22, 23]. The known risk factors for acquisition of A. baumannii infections are length of intensive care unit stay [6] stay, use of central venous catheters, antibiotic use especially extended spectrum β-lactams and fluoroquinolones, urinary catheters and comorbidities [24]. In the present study, for all patients' length of hospital stay exceeded 6 days (6 to 90 days) and 18 of them were previously hospitalized. Empiric antibiotherapy based on cephalosporin 3rd generation (N=11), amoxicillin/clavulanique acid (N=14) and imipenem (N=2) in association with aminosides or fluoroquinolones was noted in 11, 14 and 2 cases, respectively. Clinical outcome was favorable for only 1/3 of patients.
All strains were co-resistant to all β-lactams, gentamicin, amikacin, ciprofloxacin but 6 isolates remained susceptible to netilmicin, 2 isolates to tobramycin and 3 strains to trimethoprim-sulfamethoxazol. All strains were susceptible to colistin. Despite its renal toxicity, colistin has become useful antibiotic for treating infections caused by carbapenem resistant pathogens [25], but dissemination of A. baumannii resistant to colistin is worrying. In another side, many studies provide the activity of tigecycline against multidrug A. baumannii clinical isolates [26]. Antibiotic resistance in A. baumannii is frequently an interplay between several different mechanisms [5]. This bacteria produces naturally 2 intrinsic types of β-lactamases [5]. An AmpC type cephalosporinase expressed at a basal level and an oxacillinase represented by the OXA-51/69 variants [5]. When ISAba1 were inserted upstream of blaAmpC and blaOXA-51-like genes, the strains become resistant to ceftazidime and to carpenems, respectively [27, 28]. The blaOXA-51-like genes are chromosomally located in all of the A. baumannii isolates studied to date and their presence has been used to confirm identification of A. baumannii [29]. In addition to those naturally occurring β-lactamases, several acquired β-lactamases have been identified as a source of carbapenem resistance in A. baumannii. They belong to either class D (oxacillinases) or class B (metallo-β-lactamases [MBLs] [5]. The first carbapenem-hydrolysing oxacillinase was OXA-23 identified in Scotland in 1985 [30]. The gene encoding this enzyme, named ARI-1, was plasmid born and was associated with the ISAbaI transposase [30]. Since then, the IS-OXA23 structure has been found among Acinetobacter isolates from various countries [31, 32]. The bla OXA-23 genes have been identified as part of transposon structures, namely Tn2006 and Tn2007 [31]. Interestingly, the reservoir (natural producer) of this gene has been identified as being A. radioresistens [33]. This Acinetobacter species shares the same reservoir with A. baumannii, the skin flora in humans [31].
Our study revealed the presence of this carbapenemase in Tunisian (n=18) and Algerian (n=5) strains. However, blaOXA-24-like and bla OXA -58-like were not detected in any of ours strains. In the 4 strains, where only bla OXA-51 was detected, resistance can be explained by non enzymatic mechanisms or insertion of ISAba1 sequences [28, 34]. MBLs are powerful carbapenemases [35]. They have been identified worldwide in A. baumannii [36, 37]. Four groups were described in A. baumannii: IMP-like, VIM-like SIM-1 and NDM-1 enzymes [35]. MBL production was not detected in any of our strains. However, many studies describe bla NDM-1 in A. baumannii isolated from Algerian patients evacuated to France [36, 38]. Resistance to carbapenems in A. baumannii may be also due to an association between several β-lactamases and other mechanisms of resistance, such as porin(s) loss, over expression of the naturally occurring AdeABC efflux pump, and rarely modification of penicillin-binding proteins (PBPs) [39]. Nosocomial clonal diffusion of multidrug resistant A. baumannii has been reported from various regions of the world [40]. Although antibiotyping may alert to the dissemination of a multiresistant A. baumannii strain, distinguishing between strains with slight differences in their resistance profiles may be difficult. Only genotypic methods including PFGE of chromosomal DNA restriction fragments have been used to investigate nosocomial A. baumannii outbreaks [14]. Tunisian A. baumannii isolates were clustered in 6 different molecular epidemiology patterns. However, Algerian strains were clustered in one clone G.
This study shows a high distribution of blaOXA-23 in imipenem-resistant A. baumannii isolated in Tunisia and Algeria. It demonstrated the epidemic diffusion of this multidrug resistant pathogen. Thus, strengthening of prevention measures are required to control further spread of carbapenemases in the two countries.
What is known about this topic
- Acinetobacter baumannii is a nosocomial pathogen;
- It has a high ability to develop antibiotic resistance and it has become a problematic challenge in the modern healthcare system;
- The molecular and genetic mechanisms of gaining multidrug resistance in ACB complex are well known.
What this study adds
- Resistance of A.baumannii to carbapenems is mostly associated with the gene OXA-23 in Algeria and Tunisia;
- The description of the genotypic epidemiology of Acinetobacter baumannii.
The authors declare no competing interests.
Sabrina Amiri, Samia Hammami, Kamel Amoura: participated in the identification of Acinetobacter baumannii, analysis and interpretation of the phenotypic and genotypic tests, writing and critically revising manuscript. Dekhil mazouz, Boutiba Ilhem: participated in the interpretation and writing of manuscript. All authors have read and agreed to the final version of this manuscript.
This work was supported by the Ministry of Scientific Research, Technology and Competence Development of Tunisia.
Table 1: demographic and clinical characteristics of patients with carbapenem-resistant Acinetobacter baumannii
Table 2: the MICs,PCR results and PFGE clusters of the clinical isolates
Figure 1: PFGE patterns
of carbapenem resistant A. baumannii, 1-27: isolates number M: DNA lambda
ladder, (A) Pattern of Algerian isolates, (B) Pattern of Tunisian isolates
- Aghamiri S et al. Antibiotic Resistance Patterns and a Survey of Metallo-beta-Lactamase Genes Including bla-IMP and bla-VIM Types in Acinetobacter baumannii Isolated from Hospital Patients in Tehran. Chemotherapy. 2016; 61(5): 275-280. PubMed | Google Scholar
- Chastre J. Infections due to Acinetobacter baumannii in the ICU. Semin Respir Crit Care Med. 2003; 24(1): 69-78. PubMed | Google Scholar
- Neonakis IK, Spandidos DA, Petinaki E. Confronting multidrug-resistant Acinetobacter baumannii: a review. Int J Antimicrob Agents. 2011; 37(2): 102-9. PubMed | Google Scholar
- Falagas ME, Kopterides P, Siempos II. Attributable mortality of Acinetobacter baumannii infection among critically ill patients. Clin Infect Dis. 2006; 43(3): 389; author reply 389-90. PubMed | Google Scholar
- Poirel L, Nordmann P. Carbapenem resistance in Acinetobacter baumannii: mechanisms and epidemiology. Clin Microbiol Infect. 2006; 12(9): 826-36. PubMed | Google Scholar
- Gonzalez-Villoria AM, Valverde-Garduno V. Antibiotic-Resistant Acinetobacter baumannii Increasing Success Remains a Challenge as a Nosocomial Pathogen. J Pathog. 2016; 2016: 7318075. PubMed | Google Scholar
- Moradi JF, Hashemi B, Bahador A. Antibiotic Resistance of Acinetobacter baumannii in Iran: A Systemic Review of the Published Literature. Osong Public Health Res Perspect. 2015; 6(2): 79-86. PubMed | Google Scholar
- Walther-Rasmussen J, Hoiby N. OXA-type carbapenemases. J Antimicrob Chemother. 2006; 57(3): 373-83. PubMed | Google Scholar
- Walther-Rasmussen J, Hoiby N. Class A carbapenemases. J Antimicrob Chemother. 2007; 60(3): 470-82. PubMed | Google Scholar
- Evans BA, Amyes SG. OXA beta-lactamases. Clin Microbiol Rev. 2014; 27(2): p. 241-63. PubMed | Google Scholar
- Lee K et al. Multidrug-resistant Acinetobacter spp: increasingly problematic nosocomial pathogens. Yonsei Med J. 2011; 52(6): 879-91. PubMed | Google Scholar
- Opazo A et al. OXA-type carbapenemases in Acinetobacter baumannii in South America. J Infect Dev Ctries. 2012; 6(4): 311-6. PubMed | Google Scholar
- Zahedi Bialvaei A et al. Dissemination of carbapenemases producing Gram negative bacteria in the Middle East. Iran J Microbiol. 2015; 7(5): 226-46. PubMed | Google Scholar
- Yang HY et al. Outbreaks of imipenem resistant Acinetobacter baumannii producing OXA-23 beta-lactamase in a tertiary care hospital in Korea. Yonsei Med J. 2009; 50(6): 764-70. PubMed | Google Scholar
- Poirel L, Nordmann P. Acquired carbapenem-hydrolyzing beta-lactamases and their genetic support. Curr Pharm Biotechnol. 2002; 3(2): 117-27. PubMed | Google Scholar
- Hammami S et al. Carbapenem-resistant Acinetobacter baumannii producing the carbapenemase OXA-23 in Tunisia. Tunis Med. 2011; 89(7): 638-43. PubMed | Google Scholar
- Tenover FC et al. Interpreting chromosomal DNA restriction patterns produced by pulsed-field gel electrophoresis: criteria for bacterial strain typing. J Clin Microbiol. 1995; 33(9): 2233-9. PubMed | Google Scholar
- Bergogne-Berezin ETK. Acinetobacter spp as nosocomial pathogens: microbiological, clinical, and epidemiological features. Clin Microbiol Rev. 1996; 148-165. PubMed | Google Scholar
- Chastre J. Infections due to Acinetobacter baumannii in the ICU. Semin Respir Crit Care Med. 2003; 24: 69-78. PubMed | Google Scholar
- Gootz TD MA. Acinetobacter baumannii: an emerging multidrug-resistant threat. Expert Rev Anti Infect Ther. 2008; 6: 309-325. PubMed | Google Scholar
- Abbo A N-VS, Hammer-Muntz O, Krichali T, Siegman-Igra Y, Carmeli Y. Multidrug-resistant Acinetobacter baumannii. Emerg Infect Dis. 2005; 22-29. PubMed | Google Scholar
- Kim YJ et al. Risk factors for mortality in patients with carbapenem-resistant Acinetobacter baumannii bacteremia: impact of appropriate antimicrobial therapy. J Korean Med Sci. 2012; 27(5): p. 471-5. PubMed | Google Scholar
- Yang YS et al. Acinetobacter baumannii nosocomial pneumonia: is the outcome more favorable in non-ventilated than ventilated patients? BMC Infect Dis. 2013; 13: 142. Google Scholar
- Zarrilli R et al. Clonal spread and patient risk factors for acquisition of extensively drug-resistant Acinetobacter baumannii in a neonatal intensive care unit in Italy. J Hosp Infect. 2012; 82(4): 260-5. PubMed | Google Scholar
- Nordmann P, Poirel L. Emerging carbapenemases in Gram-negative aerobes. Clin Microbiol Infect. 2002; 8(6): 321-331. PubMed | Google Scholar
- Kempf M, Rolain JM. Emergence of resistance to carbapenems in Acinetobacter baumannii in Europe: clinical impact and therapeutic options. Int J Antimicrob Agents. 2012; 39(2): 105-14. PubMed | Google Scholar
- Turton JF et al. The role of ISAba1 in expression of OXA carbapenemase genes in Acinetobacter baumannii. FEMS Microbiol Lett. 2006; 258(1): 72-7. PubMed | Google Scholar
- Héritier CL, Poirel and Nordmann P. Cephalosporinase over-expression resulting from insertion of ISAba1 in Acinetobacter baumannii.Clin Microbiol Infect. 2006; 12(2): 123-30. PubMed | Google Scholar
- Carvalho KR et al. Dissemination of multidrug-resistant Acinetobacter baumannii genotypes carrying blaOXA-23 collected from hospitals in Rio de Janeiro, Brazil. Int J Antimicrob Agents. 2009; 34(1): 25-8. PubMed | Google Scholar
- Donald HM et al. Sequence analysis of ARI-1, a novel OXA beta-lactamase, responsible for imipenem resistance in Acinetobacter baumannii 6B92. Antimicrob Agents Chemother. 2000; 44(1): 196-9. PubMed | Google Scholar
- Corvec S et al. Genetics and expression of the carbapenem-hydrolyzing oxacillinase gene blaOXA-23 in Acinetobacter baumannii. Antimicrob Agents Chemother. 2007; 51(4): 1530-3. PubMed | Google Scholar
- Wang H et al. Molecular epidemiology of clinical isolates of carbapenem-resistant Acinetobacter spp from Chinese hospitals. Antimicrob Agents Chemother. 2007; 51(11): 4022-8. PubMed | Google Scholar
- Poirel L et al. Acinetobacter radioresistens as a silent source of carbapenem resistance for Acinetobacter spp. Antimicrob Agents Chemother. 2008; 52(4): 1252-6. PubMed | Google Scholar
- Romanelli RM et al. Outbreak of resistant Acinetobacter baumannii- measures and proposal for prevention and control. Braz J Infect Dis. 2009; 13(5): 341-7. PubMed | Google Scholar
- Bush K. New beta-lactamases in Gram-negative bacteria: diversity and impact on the selection of antimicrobial therapy. Clin Infect Dis. 2001; 32(7): 1085-9. PubMed | Google Scholar
- Boulanger A et al. NDM-1-producing Acinetobacter baumannii from Algeria. Antimicrob Agents Chemother. 2012; 56(4): 2214-5. PubMed | Google Scholar
- Amudhan MS et al. BlaIMP and blaVIM mediated carbapenem resistance in Pseudomonas and Acinetobacter species in India. J Infect Dev Ctries. 2012; 6(11): 757-62. PubMed | Google Scholar
- Hussenet C et al. Multidrug-resistant Acinetobacter baumannii infections in three returning travelers evacuated from Algeria, Thailand, and Turkey after hospitalization in local intensive care units. J Travel Med. 2011; 18(5): 358-60. PubMed | Google Scholar
- Gehrlein M et al. Imipenem resistance in Acinetobacter baumanii is due to altered penicillin-binding proteins. Chemotherapy. 1991; 37(6): 405-12. PubMed | Google Scholar
- Mendes RE et al. Emergence and widespread dissemination of OXA-23, -24/40 and -58 carbapenemases among Acinetobacter spp in Asia-Pacific nations: report from the SENTRY Surveillance Program. J Antimicrob Chemother. 2009; 63(1): 55-9. PubMed | Google Scholar