Analysis of Culex and Aedes mosquitoes in southwestern Nigeria revealed no West Nile virus activity
Waidi Folorunso Sule, Daniel Oladimeji Oluwayelu
Corresponding author: Waidi Folorunso Sule, Department of Biological Sciences, Faculty of Basic and Applied Sciences, Osun State University, PMB 4494, Oke-Baale, Osogbo, 230212, Osun State, Nigeria
Received: 11 Jun 2015 - Accepted: 09 Feb 2016 - Published: 17 Mar 2016
Domain: Epidemiology
Keywords: Mosquitoes, WNV-RNA, real-time RT-PCR, infection risk, southwestern Nigeria
©Waidi Folorunso Sule et al. Pan African Medical Journal (ISSN: 1937-8688). This is an Open Access article distributed under the terms of the Creative Commons Attribution International 4.0 License (https://creativecommons.org/licenses/by/4.0/), which permits unrestricted use, distribution, and reproduction in any medium, provided the original work is properly cited.
Cite this article: Waidi Folorunso Sule et al. Analysis of Culex and Aedes mosquitoes in southwestern Nigeria revealed no West Nile virus activity. Pan African Medical Journal. 2016;23:116. [doi: 10.11604/pamj.2016.23.116.7249]
Available online at: https://www.panafrican-med-journal.com//content/article/23/116/full
Original article 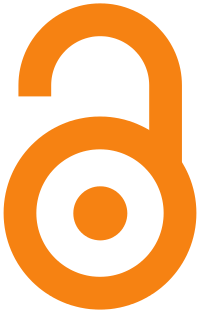
Analysis of Culex and Aedes mosquitoes in southwestern Nigeria revealed no West Nile virus activity
Analysis of Culex and Aedes mosquitoes in southwestern Nigeria revealed no West Nile virus activity
Waidi Folorunso Sule1,&, Daniel Oladimeji Oluwayelu2
1Department of Biological Sciences, Faculty of Basic and Applied Sciences, Osun State University, PMB 4494, Oke-Baale, Osogbo, 230212, Osun State, Nigeria, 2Department of Veterinary Microbiology and Parasitology, Faculty of Veterinary Medicine, University of Ibadan, Ibadan, Nigeria
&Corresponding author
Waidi Folorunso Sule, Department of Biological Sciences, Faculty of Basic and Applied Sciences, Osun State University, PMB 4494, Oke-Baale, Osogbo, 230212, Osun State, Nigeria
Introduction: amplification and transmission of West Nile virus (WNV) by mosquitoes are driven by presence and number of viraemic/susceptible avian hosts.
Methods: in order to predict risk of WNV infection to humans, we collected mosquitoes from horse stables in Lagos and Ibadan, southwestern Nigeria. The mosquitoes were sorted and tested in pools with real-time RT-PCR to detect WNV (or flavivirus) RNA using WNV-specific primers and probes, as well as, pan-flavivirus-specific primers in two-step real-time RT-PCR. Minimum infection rate (MIR) was used to estimate mosquito infection rate.
Results: only two genera of mosquitoes were caught (Culex, 98.9% and Aedes, 1.0%) totalling 4,112 females. None of the 424 mosquito pools tested was positive for WNV RNA; consequently the MIR was zero. Sequencing and BLAST analysis of amplicons detected in pan-flavivirus primer-mediated RT-PCR gave a consensus sequence of 28S rRNA of Culex quinquefasciatus suggesting integration of flaviviral RNA into mosquito genome.
Conclusion: while the latter finding requires further investigation, we conclude there was little or no risk of human infection with WNV in the study areas during sampling. There was predominance of Culex mosquito, a competent WNV vector, around horse stables in the study areas. However, mosquito surveillance needs to continue for prompt detection of WNV activity in mosquitoes.
West Nile Virus (WNV) is a mosquito-borne viral pathogen that belongs to the Flaviviridae family. It is closely related to other human pathogens such as yellow fever (YF), dengue (DEN), tick-borne encephalitis (TBE), Japanese encephalitis (JE), and Murray Valley encephalitis (MVE) viruses [1]. WNV contains a single-stranded, positive-sense RNA genome of about 11kb that is held in a nucleocapsid. The viral genome is translated as a single polyprotein, which is cleaved into three structural and seven non-structural (NS) proteins [2]. The WN virion of about 45-50 nm in diameter is contained in a host-derived membrane; the membrane has two viral glycoproteins, the membrane (M) and envelope (E) proteins, embedded in it [3].
WNV mainly infects birds but can infect many other species including humans [4]. Transmission, mainly via Culex (Cx) mosquitoes, occurs by acquisition of the virus by female mosquitoes through blood meal from infected birds (amplifying reservoir hosts) and introduction of the infectious virions to other susceptible birds and mammals during subsequent blood meals [5]. Unlike humans, horses and other mammals that are reproductive dead-end hosts [6], birds have high and durable viral titer that allows them transmit the virus to biting mosquitoes. Besides Culex spp, other mosquitoes that efficiently transmit WNV are Aedes (Ae) albopictus, Ae vexans and Ochlerotatus triseriatus [7].
WNV has become a global threat of public health and veterinary significance [8]. Thus, there is a need to conduct surveillance for the virus in mosquitoes in order to correctly provide spatio-temporal information on risk of infection in humans (and other vertebrates) [9]. WNV surveillance consists of two distinct but complementary activities: the epidemiological and environmental surveillance activities. The latter involves monitoring of local WNV activity in vectors and non-human vertebrate hosts in advance of epidemic activity that affects humans [10, 11]. WNV activity can be monitored by testing adult mosquitoes for virus infection [12] through molecular detection of viral RNA using real-time RT-PCR. This method is preferred not only for its sensitivity (detects about 0.1 PFU/ml), but also for its short turn-around time of about 4 hours [11, 13]. According to Condotta et al. [14], infection rate, which is the proportion of mosquitoes in the environment infected with virus, can be estimated using either minimum infection rate (MIR) or maximum likelihood estimate (MLE). The MIR is the number of mosquito pools infected per 1000 female mosquitoes tested and is most appropriate when < 1000 female mosquitoes are tested. Consequently, MIR of zero suggests no viral activity in study mosquitoes which implies little or no risk of human infection, MIR between 0.1 and 3.9 implies presence of some viral activity which necessitates increased vigilance and mosquito testing, while MIR of 4.0 or above indicates presence of high level of viral activity in the area and that human infections are imminent, if not already present. Compared to testing individual mosquitoes, testing sets of pooled mosquitoes of the same species is logistically the easiest and most cost-effective approach for WNV testing [14].
In Nigeria, there is no recent report of WNV isolation from mosquitoes though its RNA has been reportedly detected in mosquitoes caught in the northeastern and southwestern parts of the country [15]. In addition, considering that WNV is an RNA virus with higher propensity for mutational changes vis-à-vis climatic/environmental changes and global travels, there is a need to test mosquitoes in the rainforest southwestern Nigeria for WNV activity and viral properties with the view to predicting risk of WNV infection to humans and instituting necessary control response.
Study area
Mosquitoes were collected between June, 2013 and January, 2014 from Onosa (N06.47001°, E003.80226°) and Ajah (N06.46700°, E003.57255°) horse ranches in Lagos State, and from Eleyele Polo club (N07.4036°, E003.8726°) in Ibadan, Oyo State, both in southwestern Nigeria (Figure 1). Lagos State, with a coastline of approximately 180 km, has coastal wetlands and upland rainforest as dominant ecozones. The vegetation cover is mostly a mosaic of mangrove swamps, freshwater swamps, secondary forest, farmland and fallow land. The soils are mostly deep and poorly drained. Its climate is wet equatorial influenced by its nearness to the equator and the Gulf of Guinea. It enjoys rainy season with two peaks: May to July and September to October, with the former being the heaviest. Floods characterize the peaks due to the poor surface drainage systems of the coastal lowlands. The mean annual rainfall ranges from 1,567.2mm in the north-western part of the state to 1,750mm in the mainland areas. The temperature of the state is generally consistently high, with the mean monthly maximum temperature of about 30°C [16, 17]. The climate of Oyo State on the other hand, is typically West African Monsoon marked by distinct seasonal shifts in wind patterns. The rainy season that starts in Oyo state during the first week of March with storms averages 8 months in a year with average annual rainfall being over 1,000mm while the dry season occurs from November to February when dry, dust-laden winds blow from the Sahara desert heralding the harmattan period. The vegetation is rain forest and derived savannah, and average temperature is between 18.9°C to 35°C [18, 19]. Most of the periods of mosquito collection coincided with the wet seasons in the two states.
Study design, mosquito collection and storage
This is a mosquito surveillance study and the mosquitoes were collected using two different traps - BioGents sentinel trap (Biogents, Regensburg, Germany) and the Centers for Disease Control and Prevention (CDC) light mosquito trap (John W. Hock Company, Gainesville, FL, USA) [14, 20]. The traps are herein referred to as “BG” and “CDC” traps. The 12V battery-powered CDC and BG mosquito traps were set at the entrance of and around the horse boxes in Eleyele polo club, and at Ajah and Onosa stables. The two traps were used in order to catch as many mosquitoes as possible and to have representative catch of the mosquitoes prevalent at each location. The CDC light trap attracts most flying insects while the BG trap, equipped with Biogents Sweetscent™ attractant that simulates human body odour, mostly attracts blood-seeking mosquitoes. The traps were set from dusk (about 1800 hours) to dawn (about 0700 hours) [21]. Trapped mosquitoes in the receptacles were anaesthetized on ice packs; when they had become very weak or dead, they were packed into cryovials and transported on ice to the Virology laboratory, Department of Veterinary Microbiology and Parasitology, Faculty of Veterinary Medicine, University of Ibadan, Ibadan where they were stored at -80°C until analyzed.
Mosquito sorting, homogenization and RNA extraction
With the assistance of an entomologist and using taxonomic keys [22], the mosquitoes were sorted on ice under a stereo-microscope into pools based on location of collection, type of mosquito trap, date of collection, genus and gender. A pool contained between 1 and 12 (average of 10) adult mosquitoes of same genus. Only the females were further analyzed for presence of WNV. Each pool of female mosquitoes was homogenized using the QIAquick® PCR Purification Kit (QIAGEN, Germany) according to the manufacturer’s instructions. Briefly, two 5mm stainless steel beads were added to each vial of mosquitoes followed by addition of 600µl of lysis buffer (buffer RLT) which contains guanidine thiocyanate. The vials were tightly covered with their snap-caps and loaded into TissueLyser® (QIAGEN, Germany) already set to vibrate at 25 beats per second for 2 minutes. The homogenates were then transferred to a refrigerated centrifuge and spun at 13,200 rpm for 3 minutes. Without touching any pellet, the supernatant from each tube was dispensed into correspondingly labeled new 1.5ml tube and stored at -80°C until used for RNA extraction. RNA was extracted from each 600µl mosquito lysate using RNeasy® Mini Kit (QIAGEN, Germany) spin column according to manufacturer’s instructions. A volume of 50µl RNase-free water was used to elute the sample RNA into 1.5ml collection tube which was stored at -80°C until further analyzed.
RNA testing using One- and Two-step real-time RT-PCR
One-step real-time RT-PCR to detect WNV RNA was performed on the RNA samples with iScriptTM One-Step RT-PCR Kit (BIORAD, USA) as previously described [23]. Pipetting and plate preparation were done on ice crystals thus: 2µl RNA template was added to 48µl master mix containing 1µl iScript reverse transcriptase, 1µl each of forward and reverse primers (5pmol/µl each), 1µl WNV Linke probe (2.5pmol/µl) [24], 25µl of 2X RT-PCR reaction buffer for probes and 19µl nuclease-free water. HPLC grade water was used as no-template control (NTC) while RNA templates of WNV lineage 1 (ArB3573/82), WNV-goose Israel 1998 and WNV Lineage 2-Madagascar were included in different reactions as WNV positive controls. The sequences of primers and probes used are shown in Table 1. The reaction tubes were incubated in real-time PCR system (STRATAGENE® MX 3000P, Agilent Technologies, USA) which was programmed as follows: 50°C for 10 minutes for reverse transcription, 95°C for 5 minutes to inactivate reverse transcriptase and activate Taq polymerase, then 45 cycles of 95°C for 15 seconds and 55°C for 30 seconds for cDNA amplification. The machine was programmed to collect data for FAM. Following the One-step real-time RT-PCR using WNV-specific primers, we tested some RNA samples in two-step RT-PCR, using pan-flavivirus- and pan-alphavirus-specific primers with RNAs of WNV and louping ill virus as positive controls for pan-flavivirus reaction and those of Chikungunya and Getah viruses as positive controls for the pan-alphavirus reaction. The pan-flavivirus and pan-alphavirus primers are shown in Table 2.
For the two-step reaction, 2µl RNA template was added to 18µl master mix containing 4µl RT buffer (5X), 1µl dNTPs (10mM), 1µl DTT (0.1M), 2µl Random hexamers (50ng/µl) (Roche®), 1µl RNasin (40U/µl), 1µl MMLV-RT (200U/µl) and 8µl nuclease-free water, and incubated on heating block at 42°C for 1 hour. Thirty microliter of HPLC grade water used as NTC was added to each cooled cDNA sample to dilute it. From the latter, 5µl each was taken and added as template respectively to 35µl pan-flavivirus master mix containing 1µl Flavi-F (10pmol/µl), 1µl Flavi-R (10pmol/µl), 20µl SYBR® Green JumpStart® Taq ReadyMix®, and 13µl nuclease-free water, and to 35µl pan-alphavirus master mix containing 1µl VIR 2052F Alpha (10pmol/µl), 1µl VIR 2052R Alpha (10pmol/µl), 20µl SYBR® Green JumpStart® Taq ReadyMix®, and 13µl nuclease-free water in different reaction tubes. The cycling conditions were 95°C for 10 minutes, and 50 cycles of 95°C for 30s, 55°C for 30s and 72°C for 30s, followed by 1 cycle of 95°C for 60s, 55°C for 30s and 95°C for 30s (the machine was set to process dissociation curve starting at 72°C for 10s).
Gel electrophoresis : a 2µl aliquot of each PCR product was examined by electrophoresis on 1.8% agarose gel containing 5µl of SYBR® safe (Invitrogen, USA), PhiX174 phage DNA was used as molecular weight marker and the gel was visualized with GelDoc XR Scanner (BIORAD, USA).
Sequencing of PCR products: the amplicon from each RNA sample that gave detectable band in agarose gel electrophoresis was cleaned up using the QIAquick PCR Purification kit (QIAGEN, Germany) according to manufacturer’s protocol. The purified PCR products were subjected to nucleotide sequencing using Flavi-F: GCMATHTGGTWCATGTGG and Flavi-R: GTRTCCCAKCCDGCNGTRTC primers with ABI PRISM (r) 3100 Genetic Analyzer (Applied Biosystems Inc.).
Estimation of mosquito minimum infection rate (MIR): mosquito minimum infection rate (MIR) was estimated using the formula: (number of WNV-positive mosquito pools/total number of mosquitoes tested) x 1000 [21].
Data analysis : the results obtained were presented with descriptive statistics while nucleotide sequences generated were analyzed by performing BLAST search in the GenBank (NCBI).
In all, 4,112 female mosquitoes belonging to two genera, Culex and Aedes, were identified (Table 3). They were sorted into 424 pools comprising 413 pools of Culex (n=4,070; 98.9%) and 11 pools of Aedes (n=42; 1.0%). No male Aedes was trapped. Real-time RT-PCR of the first batch of 48 RNA samples did not give any cycle threshold (Ct) value (i.e. no detectable WNV RNA), except for a sample (N26) that gave a weird amplification plot as shown in Figure 2. The sample was a pool of 10 female Culex mosquitoes from Onosa horse stable. However, the sample (Figure 3, Lane 6) did not yield the desired band following gel electrophoresis.
The pan-flavivirus and pan-alphavirus primer-mediated two-step RT real-time PCR of sample N26 and seven others selected at random around it gave amplification plots and dissociation curves as shown in Figure 4; the pan-alphavirus reaction, however, was negative (Figure 5). Figure 6 shows the gel electrophoresis result of the samples; the pan-flavivirus reactions showed positive bands, while those of pan-alphavirus gave negative bands. The remaining 37 RNA samples also gave Ct values in pan-flavivirus reaction (data not shown).
Sequencing of the 8 samples that gave detectable RNA from the pan-flavivirus reaction (Figure 6) revealed a consensus sequence of 191 nucleotides as shown below: 5’-AAGTTGCAATATGGTACATGTGGTGATATTTAGCTTTAGAAGGAGTTTACCTCCCACTTTGTGCTGCACTATCAAGCAACA
CGACTCCATGGAAAAATTTTCCACCATCAGCACCGTCCTACGGGCCTATCACCCTCTATGGGAGTAAAAGCCACATTCTAGT
TGAACTTGGACACCGCCGGCTGGGACAC -3’.
BLAST analysis of the sequence in GenBank revealed it was identical to the 28S ribosomal RNA (rRNA) of host mosquitoes with the sequence of Culex quinquefasciatus producing significant homology. When the remaining samples were subjected to WNV primer mediated - real-time RT-PCR, only the positive controls gave Ct values while the samples were all negative (data not shown).
Minimum mosquito infection rate (MIR): estimation of MIR gave overall value of zero; the genus-, location- and trap type-specific MIRs were, of course, also zero.
This surveillance study was conducted to investigate WNV infection in mosquitoes in the rainforest ecological zone of south western Nigeria. It was noticeable that greater proportion of Culex mosquitoes - the maintenance/bridge vector in enzootic or epidemic cycle of WNV - than Aedes were collected. A probable reason for this is that the former are usually more active from dusk to dawn (night-biters) unlike Aedes that are mostly day-biting [26]. Baba et al. [15], LaBeaud et al. [21], Özer et al. [27] and Vaux et al. [28] also collected greater proportion of Culex mosquitoes in their studies.
Real-time RT-PCR for detection of WNV in mosquitoes is very sensitive and specific with short turn-around time; hence it is widely used for arboviral surveillance [8, 11]. We processed study mosquitoes according to established protocols and manufacturer’s guide and observed that the first batch of 48 RNA samples did not give any Ct values indicating presence of WNV RNA in the mosquitoes except a weird amplification plot of sample N26 which gave a Ct value of 34.93, unlike the positive WNV control with 23.39 Ct value (Figure 2). The remaining RNA samples had detectable RNA in pan-flavivirus RT-PCR but sequence analysis showed the consensus sequence as 28S rRNA of Culex quinquefasciatus. A possible explanation for this is integration of flaviviral RNA (WNV RNA inclusive) into genome of host Culex mosquitoes. Integration of flaviviral RNA (though not specifically for WNV) into host mosquito’s genome have been reported [29, 30]; we were, however, not sure whether or not this was the case in our own study. A future study is recommended to further elucidate on this.
The real-time-RT-PCR of the remaining samples did not detect any WNV RNA. Testing of mosquitoes (Culex and Aedes spp) using real-time or conventional RT-PCR without detecting WNV (or arboviral) RNA has been previously reported [8, 20, 27, 28]. The fact that WNV RNA was not detected in any of the mosquito pools tested in this study is worth noting considering that serologic studies on horse [31] and human sera (unpublished data) and previous serologic studies in humans [15, 32] showed high prevalence of anti-WNV antibodies in Nigeria. However, high anti-WNV antibody prevalence/herd immunity among amplifying host birds inversely correlated with mosquito infection rate [33, 34]. These observations might explain why, in spite of reports of WNV-specific antibodies in humans and horses, none of the tested female mosquitoes yielded WNV RNA. They might also be a possible reason for the absence of reports of WN disease outbreak or WNV-induced encephalitis in humans or horses in Nigeria.
Though we did not test birds in the study locations, the established close association between amplifying host birds and biting mosquitoes suggest that as at the time of sampling, the collected mosquitoes did not pick WNV from the local amplifying host birds during blood feeding. This could be due to absence of WN viremia or low titer viraemia caused by high prevalence of anti-WNV antibodies in the local amplifying host birds or very low number of such birds. Additionally, the caught mosquitoes were less likely to pick WNV from the sampled horses due to high antibody prevalence and presence of neutralizing antibodies [31] which have been reported to make viremia in horses or humans transient [35]. It is also possible that infectious female mosquitoes had died out, due to their short life-span (about 2 weeks for female mosquitoes), before the sampling period. Moreover, the mosquito infection rate of zero obtained in this study implies that the study mosquitoes were apparently uninfected with WNV or, there was possibility of silent undetectable WNV or other arboviral transmission cycle as noted by Roiz et al [20].
As at the time of this study, there was no detectable (active) WNV infection of mosquitoes in the study areas, at least, not at a level that could precipitate WN disease. The dominance of Culex mosquitoes, however, indicate establishment of maintenance/bridge vector of WNV in southwestern Nigeria. We suggest mosquito sampling around breeding sites of birds (resident/migratory, aquatic/terrestrial) which may yield detectable WNV RNA as well as screening of birds around the same horse stables for presence of WNV activity. Also, mosquito surveillance needs to continue for prompt detection of WNV activity in these mosquitoes.
What is known about this topic
- Mosquitoes - Cx. quinquefasciatus and mansonia sp. - were collected from northeastern and south western Nigeria by some workers with the aim of detecting WNV in them. In their study and others conducted outside Nigeria, Cx. quinquefasciatus mosquitoes, globally recognized as competent vectors of WNV, were the predominant species identified.
- While few entomologic surveillance studies for WNV and other arboviruses detected viral RNA, many of such studies reported no detection of WNV RNA.
- Some entomologic studies for WNV have reported integration of flaviviral RNA into chromosomes of Aedes host mosquitoes.
What this study adds
- This current study employed a more sensitive and specific molecular technique – the real-time RT-PCR with additional use of pan-flavivrus- and pan-alphavirus-specific primers. Also, contrary to previous study which was concluded at the gel electrophoresis step, in this study the detected amplicons from the flavivirus real-time RT-PCR were further sequenced and analyzed by BLAST search. This revealed, most likely for the first time in Nigeria, that flaviviral RNA was integrated into the host Cx. quinquefasciatus genome, unlike in Aedes sp. as previously reported. While this has considerable implication on the evolution of both the WNV and the host vector, it shows by molecular identification of 28S rRNA, that the more abundant mosquitoes were actually Cx. quinquefasciatus.
- Based on a MIR of zero, this study revealed that there was little or no risk of WNV infection to horses and humans in the study area as at the time of sampling. This highlights the need for continual entomologic surveillance to detect the period of intense WNV transmission (i.e. high risk period) in the study area.
- In line with the last observation, this study further shows the need to conduct surveillance prior to deployment of resources in order to know where and when to direct prevention or control measures/efforts.
The authors declare no competing interests.
WFS substantially contributed to conception and design of the study, he acquired the data, did the analysis and contributed to interpretation of the data; he drafted the manuscript and contributed to its revision. DOO was the originator of the study concept and contributed to its design, he contributed to data analysis and interpretation; he critiqued the drafted manuscript and contributed to its revision. Both authors approved of the final version.
We appreciate Dr. M.A. Adeleke of the Department of Biological Sciences, Osun State University, Osogbo for provision of the CDC light trap; and the support, during mosquito collection, of the grooms in the horse stables especially Mr. Danladi and Mr. Sanni (Eleyele Polo club, Ibadan) and Mr. Salisu (Ajah horse ranch, Lagos).
Table
1: genomic position and nucleotide sequences of primers and probe
for real-time RT-PCR
Table 2:
genomic positions and nucleotide sequences of primers for pan-flavivirus and
pan-alphavirus real-time PCR
Table 3: distribution of mosquito samples collected in southwestern Nigeria
Figure 1: map
of Nigeria showing southwestern states, inside which are the locations (9 as
Ibadan and 11 as Lagos) of mosquito sampling (www.fao.org_wairdocs_ilri_x5458e_x5458e0a.gif)
Figure 2: real-time amplification of 48 RNA samples using WNV Linke primers and probe
Figure 3: RT-PCR detection
of 6 RNA samples from 6 pools of female mosquitoes. Lanes 1and 10: Molecular
weight markers, Lanes 2 – 7: Test samples, Lane 8: No template control,
Lane 9: positive control
Figure 4: two-step RT-qPCR amplification plots of 8 RNA samples using pan-flavivirus primers and the corresponding dissociation curves
Figure 5: two-step RT-qPCR amplification plots of the 8 RNA samples using pan-alphavirus primers and their dissociation curves
Figure 6: gel
detection of the 8 RNA samples from two-step RT-qPCR of pan-flavivirus and pan-alphavirus
reactions: lanes 1, 13 and 25: Molecular weight markers, lanes 2 – 9: Test
samples, lanes 10 and 22: No template controls, lanes 11, 12, 23 and 24: positive
controls, lanes 14-21: test samples
- Chung, KM, Liszewski MK, Nybakken G, Davis AE, Townsend RR, Fremont DH, Atkinson JP, Diamond MS. West Nile virus nonstructural protein NS1 inhibits complement activation by binding the regulatory protein factor H. Proc Natl Acad Sci USA. 2006 Dec 12;103(50):19111-6. PubMed | Google Scholar
- Chambers TJ, Hahn CS, Galler R, Rice CM. Flavivirus genome organization, expression, and replication. Annu Rev Microbiol. 1990;44:649-88. PubMed | Google Scholar
- Schmidt K. Cellular factors modulating the entry efficiency of West Nile virus – Involvement of integrins. Ph.D thesis, University of Veterinary Medicine Hannover, Germany. 2012; pp 226. Google Scholar
- Brandler S, Tangy F. Vaccines in Development against. West Nile Virus. Viruses. 2013 Sep 30;5(10):2384-409. PubMed | Google Scholar
- Ciota AT, Kramer LD. Vector-Virus Interactions and Transmission Dynamics of West Nile Virus. Viruses. 2013 Dec 9;5(12):3021-47. PubMed | Google Scholar
- Beasley DW, Whiteman MC, Zhang S, Huang CY, Schneider BS, Smith DR, Gromowski GD, Higgs S, Kinney RM, Barrett AD. Envelope protein glycosylation status influences mouse neuroinvasion phenotype of genetic lineage 1 West Nile virus strains. J Virol. 2005 Jul;79(13):8339-47. PubMed | Google Scholar
- Centre for Food Security and Public health [CFSPH]. West Nile Virus Infection. 2009; Available at: www.cfsph.iastate.edu/IICAB/. Google Scholar
- Engler O, Savini G, Papa A, Figuerola J, Groschup MH, Kampen H, Medlock J, Vaux A, Wilson AJ, Werner D, et al. European Surveillance for West Nile Virus in Mosquito Populations. Int J Environ Res Public Health. 2013 Oct 11;10(10):4869-95. PubMed | Google Scholar
- Kilpatrick AM, Pape WJ. Predicting Human West Nile Virus Infections with Mosquito Surveillance Data. Am J Epidemiol. 2013; 178(5):829-835. PubMed | Google Scholar
- Lindsey NP, Kuhn S, Campbell GL, Hayes EB. West Nile virus neuroinvasive disease incidence in the United States, 2002-2006. Vector-Borne Zoonotic Dis. 2008; 8(1):35-40. PubMed | Google Scholar
- Centers for Disease Control and Prevention. West Nile Virus in the United States: Guidelines for Surveillance, Prevention, and Control. 4th Revision, Division of Vector-Borne Diseases, Fort Collins, Colorado, USA. 2013; pp 1-69. Available at: www.cdc.gov/westnile/resources/pdfs/wnvguidelines.pdf. Google Scholar
- Brown Jr EG. California mosquito-borne virus surveillance & response plan. Vector-Borne Disease Section, California Department of Public Health, Mosquito & Vector Control Association of California University of California. 2012; Available at: http://westnile.ca.gov. Google Scholar
- Medina GE, Sandoval EA, Rentería TB, López G, De la Mora A, Pujol LC. West Nile Virus detection by RT-PCR from mosquitoes in a locality of Baja California, Mexico. Rev Latinoam Microbiol. 2008; 50 (3-4):83-86. PubMed | Google Scholar
- Condotta SA, Hunter FF, Bidochka MJ. West Nile Virus Infection Rates in Pooled and Individual Mosquito Samples. Vector-Borne Zoonotic Dis. 2004; 4(3): 198-208. PubMed | Google Scholar
- Baba MM, Saron M-F, Diop O, Mathiot C, Adeniji JA, Olaleye OD. West Nile Virus in Mosquitoes and Febrile Patients in a Semi-arid Zone, Nigeria. J Am Sc. 2006; 2(2):28-34. PubMed | Google Scholar
- Iwugo KO, D’Arcy B, Andoh R. Aspects of Land-based Pollution of an African Coastal Megacity of Lagos. Poster Paper 14 -122. Diffuse Pollution Conference, Dublin, 2003. Google Scholar
- Building Nigeria's Response to Climate Change (BNRCC) Project. 2012. Towards a Lagos State Climate Change Adaptation Strategy (LAS-CCAS). ISBN 978-0-9878656-1-8. pp 2-5. Google Scholar
- Ajayi O, Agbola SB, Olokesusi BF, Wahab B, Taiwo OJ, Gbadegesin M, Taiwo DO, Kolawole O, Muili A, Adeola MA, et al. 2012. Flood Management in an Urban Setting: A Case Study of Ibadan Metropolis. Hydrology for Disaster Management Special Publication of the Nigerian Association of Hydrological Sciences; Available at: http://www.unaab.edu.ng. Google Scholar
- Ganiyu MO, Akinniran TN, Adeyemo SA. 2013 Rainfall Pattern and Trend on Arable Crops Production in Oyo State, Nigeria (1990-2009). World Rural Observations. 2013;5(2):7-11. PubMed | Google Scholar
- Roiz D, Vazquez A, Rosà R, Muñoz J, Arnoldi D, Rosso F, Figuerola J, Tenorio A, Rizzoli A. Blood meal analysis, flavivirus screening, and influence of meteorological variables on the dynamics of potential mosquito vectors of West Nile virus in northern Italy. Journal of Vector Ecology. 2012;37(1):20-28. PubMed | Google Scholar
- LaBeaud AD, Sutherland LJ, Muiruri S, Muchiri EM, Gray LR, Zimmerman PA, Hise AG, King CH. Arbovirus Prevalence in Mosquitoes, Kenya. Emerg Infect Dis. 2011;17(2):233-241. PubMed | Google Scholar
- Service MW. Medical entomology for students. 3rd Edition. Cambridge University Press, UK, 2004; 273pp. Google Scholar
- Johnson N, Wakeley PR, Mansfield KL, McCracken F, Haxton B, Phipps LP, Fooks AR. Assessment of a Novel Real-Time Pan-Flavivirus RT-Polymerase Chain Reaction. Vector-Borne Zoonotic Dis. 2010; 10(7):665-671. PubMed | Google Scholar
- Linke S, Ellerbrok H, Niedrig M, Nitsche A, Pauli G. Detection of West Nile virus lineages 1 and 2 by real-time PCR. J Virol Methods. 2007; 146(1-2):355-358. PubMed | Google Scholar
- Eshoo MW, Whitehouse CA, Zoll ST, Massire C, Pennella T-TD, Blyn LB, Sampath R, Hall TA, Ecker JA, Desai A et al. Direct broad-range detection of alphaviruses in mosquito extracts. Virology. 2007 Nov 25;368(2):286-95. PubMed | Google Scholar
- World Health Organization. Mosquitos and other biting Diptera, In Vector Control - Methods for Use by Individuals and Communities. Geneva, ISBN 92 4 154494 5. 1997; p 6-28. Google Scholar
- Özer N, Ergünay K, Simsek F, Kaynas S, Alten B, Caglar SS, Ustacelebi S. West Nile virus studies in the Sanliurfa Province of Turkey. Journal of Vector Ecology. 2007;32(2):202-206. PubMed | Google Scholar
- Vaux AGC, Gibson G, Hernandez-Triana LM, Cheke RA, McCracken F, Jeffries CL, Horton DL, Springate S, Johnson N, Fooks AR, Leach S, Medlock JM. Enhanced West Nile virus surveillance in the North Kent marshes, UK. Parasites and Vectors. 2015; 8:91. PubMed | Google Scholar
- Crochu S, Shelley CS, Attoui H, Charrel RN, De Chesse R, Belhouchet M, Lemasson J-J, de Micco P, de Lamballerie X. Sequences of flavivirus-related RNA viruses persist in DNA form integrated in the genome of Aedes spp Mosquitoes. J Gen Virol. 2004 Jul;85(Pt 7):1971-80. PubMed | Google Scholar
- Roiz D, Vázquez A, Seco MPS, Tenorio A, Rizzoli A. Detection of novel insect flavivirus sequences integrated in Aedes albopictus (Diptera: Culicidae) in Northern Italy. Virology Journal. 2009; 6:93. PubMed | Google Scholar
- Sule WF, Oluwayelu DO, Adedokun RAM, Rufai N, McCracken F, Mansfield KL, Johnson N. High Seroprevelance of West Nile Virus Antibodies Observed in Horses from Southwestern Nigeria. Vector-Borne Zoonotic Dis. 2015;15(3):218-220. PubMed | Google Scholar
- Baba M, Logue CH, Oderinde B, Abdulmaleek H, Williams J, Lewis J et al. Evidence of arbovirus co-infection in suspected febrile malaria and typhoid patients in Nigeria. J Infect Dev Ctries. 2013; 7(1):051-059. PubMed | Google Scholar
- Kwan JL, Kluh S, Reisen WK. Antecedent Avian Immunity Limits Tangential Transmission of West Nile Virus to Humans. PLoS ONE. 2012;7(3):e3412. PubMed | Google Scholar
- McKee E M. West Nile Virus Seroreversion and the Influence of Herd Immunity on Disease Risk in a Long-term Study of Free-ranging Birds. Student Theses. 2012; Paper 9. Available at: http://opus.govst.edu/theses. Google Scholar
- Solomon T, Ooi MM, Beasley DWC, Mallewa M. West Nile encephalitis. Br Med J. 2003 Apr 19;326(7394):865-9. PubMed | Google Scholar