High prevalence of methicillin resistant staphylococci strains isolated from surgical site infections in Kinshasa
Jean-Marie Liesse Iyamba, José Mulwahali Wambale, Cyprien Mbundu Lukukula, Ntondo za Balega Takaisi-Kikuni
Corresponding author: Jean-Marie Liesse Iyamba, Laboratory of Experimental and Pharmaceutical Microbiology, Faculty of Pharmaceutical Sciences, University of Kinshasa, Democratic Republic of Congo
Received: 23 Apr 2014 - Accepted: 25 Jun 2014 - Published: 21 Aug 2014
Domain: Public Health
Keywords: Methicillin resistant staphylococci, antibiotic sensitivity, surgical site infections, Kinshasa
©Jean-Marie Liesse Iyamba et al. Pan African Medical Journal (ISSN: 1937-8688). This is an Open Access article distributed under the terms of the Creative Commons Attribution International 4.0 License (https://creativecommons.org/licenses/by/4.0/), which permits unrestricted use, distribution, and reproduction in any medium, provided the original work is properly cited.
Cite this article: Jean-Marie Liesse Iyamba et al. High prevalence of methicillin resistant staphylococci strains isolated from surgical site infections in Kinshasa. Pan African Medical Journal. 2014;18:322. [doi: 10.11604/pamj.2014.18.322.4440]
Available online at: https://www.panafrican-med-journal.com//content/article/18/322/full
Original article 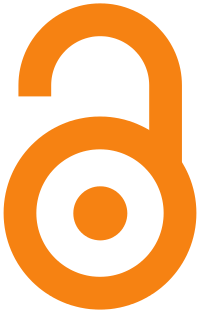
High prevalence of methicillin resistant staphylococci strains isolated from surgical site infections in Kinshasa
High prevalence of methicillin resistant staphylococci strains isolated from surgical site infections in Kinshasa
Jean-Marie Liesse Iyamba1,&, José Mulwahali Wambale1, Cyprien Mbundu Lukukula1, Ntondo za Balega Takaisi-Kikuni1
1Laboratory of Experimental and Pharmaceutical Microbiology, Faculty of Pharmaceutical Sciences, University of Kinshasa, Democratic Republic of Congo
&Corresponding author
Jean-Marie Liesse Iyamba, Laboratory of Experimental and Pharmaceutical Microbiology, Faculty of Pharmaceutical Sciences, University of Kinshasa, Democratic Republic of Congo
Introduction: Surgical site infections (SSIs) after surgery are usually caused by Staphylococcus aureus and coagulase-negative staphylococci (CNS). In low income countries, methicillin resistant Staphylococcus aureus (MRSA) and methicillin resistant coagulase-negative staphylococci (MR-CNS) surgical site infections are particularly associated with high treatment cost and remain a source of mortality and morbidity. This study aimed to determine the prevalence and the sensitivity to antibiotics of MRSA and MR-CNS isolated from SSIs.
Methods: Wound swabs were collected from 130 hospitalized surgical patients in two major hospitals of Kinshasa. S. aureus and CNS strains were identified by standard microbiological methods and latex agglutination test (Pastorex Staph-Plus). The antibiotic susceptibility of all staphylococcal strains was carried out using disk-diffusion method.
Results: Eighty nine staphylococcal strains were isolated. Out of 74 S. aureus and 15 CNS isolated, 47 (63.5%) and 9 (60%) were identified as MRSA and MR-CNS respectively. Among the MRSA strains, 47 strains (100%) were sensitive to imipenem, 39 strains (89%) to amoxycillin-clavulanic acid and 38 strains (81%) to vancomycin. All MR-CNS were sensitive to imipenem, amoxycillin-clavulanic acid and vancomycin. The isolated MRSA and MR-CNS strains showed multidrug resistance. They were both resistant to ampicillin, cotrimoxazole, erythromycin, clindamycin, ciprofloxacin, cefotaxime and ceftazidime.
Conclusion: The results of the present study showed a high prevalence of MRSA and MR-CNS. Imipenem, amoxycillin-clavulanic acid and vancomycin were the most active antibiotics. This study suggests that antibiotic surveillance policy should become national priority as MRSA and MR-CNS were found to be multidrug resistant.
Patients that are undergoing surgery or surgical procedures are at risk of acquiring infections at the site of incision. Surgical site infections (SSIs) are defined as infections occurring up to 30 days after surgery (or up to one year after surgery in patients receiving implants) and affecting either the incision or deep tissue at the operation site [1]. Despite improvements in prevention, SSIs remain a significant clinical problem as they are associated with substantial mortality and morbidity and impose severe demands on healthcare resources [2]. Microbiology studies on SSIs have shown that most of them are caused by skin-derived bacteria such as Staphylococcus aureus and coagulase-negative staphylococci [2, 3]. These bacteria, particularly methicillin resistant Staphylococcus aureus (MRSA) and methicillin resistant-coagulase negative staphylococci (MR-CNS) are known for they ability to develop resistance to multiple antibiotics [4, 5]. Studies on antimicrobial resistance of staphylococci and other bacteria isolated from hospitalized surgical patients were conducted in some African countries [6-9]. But in the Democratic Republic of Congo (DRC), the resistance pattern of Staphylococcus, especially MRSA and MR-CNS from SSIs to antibiotics remains unknown due to lack of antibiotic surveillance policies. The objective of the present study was to determine the prevalence and antibiotic susceptibility patterns of MRSA and MR-CNS isolated from SSIs in two major hospitals of Kinshasa.
Origin of staphylococci strains and laboratory procedure
This study was conducted between January to October 2013 in the Provincial General Reference Hospital of Kinshasa (HGPRK) and in Clinic of University of Kinshasa (CUK), a tertiary hospital structure. The clinical samples were collected for diagnostic purposes by the bacteriology laboratories of these hospitals and were from purulent lesions of infected sites of 130 hospitalized surgical patients. Infected sites were aseptically cleaned using normal saline and sterile gauzes. Then a wound swab from each patient was collected using sterile cotton swabs in Stuart transport media (Difco, BD Franklin Lakes, NJ, USA) and was processed in the microbiology laboratory of the Faculty of Pharmaceutical Sciences, University of Kinshasa.
Isolation and Identification of staphylococci
Wound swabs were inoculated into mannitol-salt agar (Liofilchen, Roseto degli Abbruzzi, Italy) and incubated at 37°C for 24 hours. The genera Staphylococcus was confirmed using Gram staining and catalase reaction. The identification of Staphylococcus aureus strains was performed with latex agglutination test (Pastorex Staph-Plus, BioRad, Marnes-la-Coquette, France) and DNase test. All staphylococcal strains, negative for latex agglutination and DNase tests, were considered as coagulase negative staphylococci.
Antibiotic susceptibility tests
Antibiograms of each isolated strains using the diffusion method on Mueller Hinton Agar were realized with the following antibiotic disks (Liofilchen, Roseto degli Abruzzi, Italy): amoxicillin-clavulanic acid (30 µg), ampicillin (10 µg), ciprofloxacin (5 µg), cefotaxime (30 µg), ceftazidime (30 µg), clindamycin (2 µg), erythromycin (15 µg), imipenem (10 µg), cotrimoxazole (25 µg), and vancomycin (30 µg). Test for methicillin resistance was performed with diffusion method using oxacillin (1 µg) on Mueller Hinton agar with 4 % NaCl. Evaluation of the results was done according to the criteria of Clinical Laboratory Standards Institute (CLSI) [10]. S. aureus ATCC 25923 and Staphylococcus epidermidis ATCC 12228 were used for quality control.
Eighty-nine staphylococci were isolated during the period of the study. Seventy-four strains were S. aureus and 15 strains were CNS. The S. aureus and CNS strains demonstrated good sensitivity patterns to imipenem, amoxicillin-clavulanic acid, and vancomycin. However, most S. aureus and CNS strains were resistant to ampicillin, cotrimoxazole, ciprofloxacin, erytromycin, clindamycin and ceftazidime (Table 1, Table 2). Out of the 74 S. aureus strains isolated, 47 (63.5%) were MRSA (Table 3). All these strains were sensitive to imipenem, 39 strains (89%) to amoxicillin-clavulanic acid and 38 strains (81%) to vancomycin. Nine (60%) of 15 CNS strains were MR-CNS (Table 4). All MR-CNS strains were fully sensitive to amoxicillin-clavulanic acid, imipenem, and vancomycin. The MRSA and MR-CNS strains demonstrated a high level of resistance to ampicillin, cotrimoxazole, erythromycin, clindamycin, ciprofloxacin, cefotaxime and ceftazidime. Multidrug resistance was observed in MRSA and MR-CNS strains (Table 5).
The results obtained in the present study demonstrated high prevalence of MRSA (63.5%) and MR-CNS (60%). This prevalence is higher in comparison with other studies conducted in some African countries. Report from Uganda demonstrated that the proportion of MRSA among S. aureus strains isolated from SSIs was 37.5% [6]. A survey conducted in Mulago National Referral Hospital (Uganda) on the surgical inpatients showed also an MRSA prevalence of 42% [11]. However, our results are not consistent with those obtained in Bobo Dioulasso and Nairobi where very low MRSA prevalence was observed [7, 12]. Screening of MR-CNS isolates showed a high prevalence rate. Very few African studies have been focused on the prevalence of CNS SSIs [13]. However, the current results are consistent with report from Switzerland in which the MR-CNS rate increased from 20% at the admission to 47% after surgery [14]. The highest MRSA and MR-CNS prevalence observed in our study can be attributed to the high pressure use of broad spectrum antibiotics or to the long stay hospital [15, 16]. In general, MRSA is known to be transmitted mostly by health-care contacts [17, 18]. A Congolese study among nasal staphylococcal carriers reported an MRSA prevalence of 63.1%, 66.7% and 23.3% in hospitalized patients, hospital staff team and outpatients, respectively [19]. Genomic characterization studies should be conducted in order to determine different genotypes of Staphylococus aureus strains circulating among SSIs patients and hospital staff. The MRSA and MR-CNS strains were generally multidrug resistant with an increasing resistance to ampicillin, cotrimoxazole, erythromycin, clindamycin, ciprofloxacin, ceftazidime and cefotaxime. This observation is in line with previous studies [20, 21]. The multidrug resistance observed is probably due to the ability of MRSA and MR-CNS strains to produce biofilms. Staphylococci are known as especially good biofilm formers, which is due primarily to the production of a series of surface molecules that promote extracellular matrix formation [22]. Colonization of a biotic surface such as wound by S. aureus has been demonstrated in vivo in pigs and the biofilms formed were less susceptible to antimicrobials than the planktonic counterparts [23].
A report from Italy also showed that S. aureus strains isolated from SSIs and producing a biofilm were highly resistant to antibiotic treatment [24]. This observation suggests that bacteria within the wound or the surgical lesion are encased in an extracellular polymeric matrix, change their phenotype and become recalcitrant to antibiotics and to host defenses [22]. None MR-CNS strain was resistant to amoxicillin-clavulanic acid, imipenem and vancomycine. We observed in the present study rates of Vancomycin Resistant S. aureus (VRSA) of 15 % in all S. aureus strains and 19% in MRSA strains. Our findings were consistent with a previous report from Libya in which MRSA collected from different clinical specimens showed a VRSA prevalence of 17%, using disc-diffusion method [25]. The increase of VRSA prevalence could be explained not only by the long use of vancomycin, but also by an infection or concurrent colonization with vancomycin resistant enterococci [26].
The results of the present study indicate that amoxicillin-clavulanic acid, imipenem and vancomycine should be recommended in the treatment of SSIs involving MRSA and MR-CNS. But, considering the emergence of multidrug resistant MRSA and MR-CNS strains, antibiotic susceptibility testing should be done prior to treatment in S. aureus and CNS surgical site infections.
The authors declare no competing interest.
Jean-Marie Liesse Iyamba contributed to the conception of the study, the analysis of the results and the preparation of the manuscript. Ntondo za Balega Takaisi-Kikuni contributed to the conception of the study and the preparation of manuscript. José Mulwahali Wambale contributed to the preparation of the manuscript. Cyprien Mbundu Lukukula contributed to the processing of samples. All the authors have read and approved the final version of the manuscript.
We thank members of hospital staff teams of the General Reference Hospital of Kinshasa (HGPRK) and the Clinic of University of Kinshasa (CUK), Kinshasa, DRC for their precious help during the period of study.
Table 1: Antibiotic susceptibility patterns of all S. aureus strains
Table 2: Antibiotic susceptibility patterns of all coagulase negative staphylococci strains
Table 3: Antibiotic susceptibility patterns of MRSA strains
Table 4: Antibiotic susceptibility patterns of MR-CNS strains
Table 5: Different resistance patterns of MRSA and MR-CNS strains
- Horan TC, Gaynes RP, Martone WJ, Jarvis WR, Emori TG. CDC definitions of nosocomial surgical site infections, 1992: a modification of CDC definitions of surgical wound infections. Infect Control Hosp Epidemiol. 1992; 13(10): 606-608. PubMed | Google Scholar
- Owens CD, Stoessel K. Surgical site infections: epidemiology, microbiology and prevention. J Hosp Infect. 2008; 70(S2):3-10. PubMed | Google Scholar
- Dohmen PM. Antibiotic resistance in common pathogens reinforces the need to minimise surgical site infections. J Hosp Infect. 2008; 70(S2):15-20. PubMed | Google Scholar
- Breurec S, Fall C, Pouillot R, Boisier P, Brisse S, Diene-Sarr F, Djibo S, et al. Epidemiology of methicillin-resistant Staphylococcus aureus lineages in five major African towns: emergence and spread of atypical clones. Clin Microbiol Infect. 2011; 17(4): 160-165. PubMed | Google Scholar
- Rahman A, Hossain MA, Paul SK, Sultana S, Haque N, Kabir MR, Hoque SM. Methicillin-resistant coagulase-negative staphylococci (MRCoNS) by disk diffusion method. Mymensingh Med J. 2013; 22(2):229-31. PubMed | Google Scholar
- Seni J, Bwanga F, Najjuka CF, Makobore P, Okee M, et al. Molecular characterization of Staphylococcus aureus from patients with surgical site infections at Mulago Hospital in Kampala, Uganda. PLoS One. 2013; 8(6):e66153. PubMed | Google Scholar
- Dinda V, Gunturu R, Kariuki S, Hakeem A, Raja A, Kimang'a A. Pattern of pathogens and their sensitivity isolated from surgical site infections at the Aga Khan University Hospital, Nairobi, Kenya. Ethiop J Health Sci. 2013; 23(2):141-9. PubMed | Google Scholar
- Jido T, Garba I. Surgical-site Infection Following Cesarean Section in Kano, Nigeria. Ann Med Health Sci Res. 2012; 2(1):33-6. PubMed | Google Scholar
- Eriksen HM, Chungulu S, Kondo S, Lingaas E. Surgical-site infections at Kilimanjaro Christian Medical Center. J Hosp Infect. 2003; 55(1):14-20. PubMed | Google Scholar
- CLSI. Perfomance standars for antimicrobial susceptibility testing; twenty first information supplement- CLSI document M100-S21. Wayne, PA: Clinical and Laboratory Standards Institute. 2011. PubMed | Google Scholar
- Kitara LD, Anywar AD, Acullu D, Odongo-Aginya E, Aloyo J, Fendu M. Antibiotic susceptibility of Staphylococcus aureus in suppurative lesions in Lacor Hospital, Uganda. Afr Health Sci. 2011; 11 (S1):S34-9. PubMed | Google Scholar
- Ouédraogo AS, Somé DA, Dakouré PW, Sanon BG, Birba E, et al Bacterial profile of surgical site infections at Souro Sanou National Hospital Center in Bobo Dioulasso, Burkina Faso. Med Trop. 2011; 71(1):49-52. PubMed | Google Scholar
- Melaku S, Gebre-Selassie S, Damtie M, Alamrew K. Hospital acquired infections among surgical, gynaecology and obstetrics patients in Felege-Hiwot referral hospital, Bahir Dar, northwest Ethiopia. Ethiop Med J. 2012; 50(2):135-44. PubMed | Google Scholar
- Zingg W, Demartines N, Imhof A, Senn G, Ruef C. Rapid colonization with methicillin-resistant coagulase-negative staphylococci after surgery. World J Surg. 2009; 33(10):2058-62. PubMed | Google Scholar
- Taconnelli E, De Angelis G, Cataldo MA, Pozzi E, Cauda R. Does antibiotic increase the risk of methicillin-resistant Staphylococcus aureus ? A systematic revieuw and meta-analysis. J Antimicrob Chemother. 2008; 61(1): 26-38. PubMed | Google Scholar
- Catry B, Latour K, Jans B, Vandendriessche S, Preal R, Mertens K, Denis O. Risk factors for methicillin resistant Staphylococcus aureus: A multi-laboratory study. PLoS One. 2014; 9(2):e89579. PubMed | Google Scholar
- Grundmann H, Hori S, Winter B, Tami A, Austin DJ. Risk factors for the transmission of methicillin-resistant Staphylococcus aureus in an adult intensive care unit: fitting a model to the data. J Infect Dis. 2002; 185:481?8. PubMed | Google Scholar
- Thompson RL, Cabezudo I, Wenzel RP. Epidemiology of nosocomial infections caused by methicillin-resistant Staphylococcus aureus. Ann Intern Med. 1982; 97:309-17. PubMed | Google Scholar
- Vlieghe E, Phoba MF, Muyembe Tamfun JJ, Jacobs J. Antibiotic resistance among bacterial pathogens in Central Africa: a review of the published literature between 1955 and 2008. Int J Antimicrob Agents. 2009; 34(4):295-303. PubMed | Google Scholar
- Ojulong J, Mwambu TP, Joloba M, Bwanga F, Kaddu-Mulindwa DH. Relative prevalence of methicilline resistant Staphylococcus aureus and its susceptibility pattern in Mulago Hospital, Kampala, Uganda. Tanzan J Health Res. 2009; 11(3):149-53. PubMed | Google Scholar
- Seni J, Najjuka CF, Kateete DP, Makobore P, Joloba ML, et al. Antimicrobial resistance in hospitalized surgical patients: a silently emerging public health concern in Uganda. BMC Res Notes. 2013; 6:298. PubMed | Google Scholar
- Otto M. Staphylococcal biofilms. Curr Top Microbiol Immunol. 2008; 322: 207-228. PubMed | Google Scholar
- Davis SC, Ricotti C, Cazzaniga A, Welsh E, Eaglstein WH, Mertz PM. Microscopic and physiologic evidence for biofilm-associated wound colonization in vivo. Wound Repair Regen. 2008; 16(1):23 - 29. PubMed | Google Scholar
- Pulcrano G, Vollara A, Rossano F, Catania MR. Molecular and phenotypic characterization of methicillin-resistant Staphylococcus aureus from surgical site infections. Surg Infect. 2013; 14(2): 196-202. PubMed | Google Scholar
- Buzaid N, Elzouki AN, Taher I, Ghenghesh KS. Methicillin-resistant Staphylococcus aureus (MRSA) in a tertiary surgical and trauma hospital in Benghazi, Libya. J Infect Dev Ctries. 2011; 5(10): 723-726. PubMed | Google Scholar
- Cosgrove SE, Carroll KC, Perl TM. Staphylococcus aureus with reduced susceptibility to vancomycin. Clin Infect Dis. 2004;39(4):539-45. PubMed | Google Scholar